Retina specialists often think of optical coherence tomography angiography (OCTA) as a replacement for fluorescein angiography (FA) and indocyanine green angiography (ICGA), but it’s so much more. I performed very few dye-based angiographic procedures prior to adopting OCTA, so the use of OCTA in lieu of FA and ICGA had very little impact on my practice. Instead, I found that OCTA added a whole new dimension to my clinical practice and has provided unique insights into disease diagnosis and management.
OCTA offers the value of three-dimensional structural imaging of the macula along with providing one the ability to view normal and pathologic blood vessels. By obtaining these high-density and three-dimensional macula images, I developed a greater appreciation of how neovascularization starts, even before exudation occurs; how neovascularization responds to treatment; and how I can improve my treatment regimens by following the size of the neovascularization rather than just the presence of exudation. OCTA provides a better understanding of how the disease and anti-VEGF therapy affect the macular microvasculature. As a result, I can understand my patients’ symptoms and how best to manage their disease.
WHAT IS OCTA?
We can perform OCTA using two different technologies: spectral-domain OCTA (SD-OCTA) and swept-source OCTA (SS-OCTA). These technologies image blood vessels by performing repeated OCT B-scans in the exact same position on the macula. We obtain this set of multiple B-scans repeatedly, with each set several microns away from the previous set. The size of this raster scan array and the algorithm used to detect blood flow will differ between instruments. The algorithms used to detect blood flow compare the B-scans performed at the same position, and due to the scattering properties of the flowing erythrocytes, the algorithms use changes in the intensity or phase information between the rapidly repeated scans to generate a decorrelation flow signal.
Because only erythrocytes are in motion, and the other surrounding tissue is static, these flow signals represent the perfused vasculature and can be shown on a B-scan or as an en face image to depict the microvasculature, much like we would view conventional angiography. However, the OCTA algorithms can only detect blood vessels that contain flowing erythrocytes. If there’s no flowing blood or the blood is too slow to detect, then we do not see the vessels. Therefore, we can never say the vessels are not perfused or absent, we can only say that the perfusion, if present, cannot be detected. Moreover, the added benefit of en face OCTA imaging is that we can obtain depth-resolved flow information to slice the retina and choroid through different layers and obtain layer-specific flow information.
SPECTRAL-DOMAIN VS. SWEPT-SOURCE OCTA
The biggest difference between SD-OCTA and SS-OCTA lies in the light source. With SD-OCTA, the light source has a center wavelength of 840 nm, whereas SS-OCTA has a center wavelength of ~1050 nm. This difference in wavelength influences how well the light penetrates through the retinal pigment epithelium and into the choroid. Moreover, the SS-OCTA technology also exhibits less sensitivity roll-off, which means less signal is lost as the light penetrates deeper into the back of the eye. Overall, SS-OCTA should result in better imaging of choroidal vessels and choroidal neovascularization (CNV).
In a prospective OCT imaging study published this year in Investigative Ophthalmology & Visual Science, my colleagues and I compared imaging of CNV using SS-OCTA and SD-OCTA. We collected 3 mm × 3 mm and 6 mm × 6 mm scans with a 100-kHz SS-OCT instrument and a 68-kHz SD-OCTA instrument (both Carl Zeiss Meditec).1 In this study, we used the same algorithms and segmentation analyses to analyze the scans from both instruments.
We repeated B-scans four times at each position in the 3 mm × 3 mm scans and twice in the 6 mm × 6 mm scans. For the 3 mm × 3 mm scans, the mean lesion areas for the SS-OCTA and SD-OCTA instruments were 1.17 mm2 and 1.01 mm2, respectively (P=.047). For the 6 mm × 6 mm scans, the mean lesion areas for the SS-OCTA and SD-OCTA instruments were 1.24 mm2 and 0.74 mm2, respectively (P=.003). We found that the areas of CNV were larger when imaged with SS-OCTA than with SD-OCTA, and this difference was greater for the 6 mm × 6 mm scans. In conclusion, we assessed that the SS-OCTA imaging provided a more accurate representation of the CNV compared with SD-OCTA imaging. This is important because neovascularization tends to grow at the margins of the lesion; SS-OCTA was better at identifying this lesion growth compared with SD-OCTA. It is this lesion growth that serves as a harbinger of exudation in patients receiving anti-VEGF therapy for CNV secondary to AMD. Better detection of lesion growth and better timing of treatment should result in better long-term visual acuity in these patients, but this needs to be studied formally in a clinical trial. Another issue to keep in mind is that SS-OCTA is more expensive.
The other difference between SD-OCTA and SS-OCTA is the scanning rate or the speed of the instrument. Most SD-OCTA instruments scan at about 70,000 A-scans per second, whereas the SS-OCTA instrument scans at a starting rate of 100,000 A-scans per second, with much faster rates possible.2 An increased scanning rate allows for a larger area to be scanned for a given chair time. The shorter the acquisition time, the more cooperative a patient will be compared with a slower scanning rate and a longer acquisition time.
OCTA’S IMPACT
Structural OCT images have provided me with most of the information necessary for the routine diagnosis and management of my patients with macular diseases. But I have found that OCTA removes almost all diagnostic uncertainty and improves disease management for patients who have both nonproliferative and proliferative diabetic retinopathy (DR), AMD and retinal vein occlusions (RVOs). For example, with SS-OCTA, we can visualize the development of neovascularization in patients with dry AMD for weeks or months before they become symptomatic. We can also reliably detect type 1, 2 and 3 lesions in patients with neovascular AMD, and detect subtle neovascularization in proliferative DR and RVOs.
Once we identify these lesions, we can use OCTA to fine-tune management by measuring the lesions’ size and growth as we treat those with diabetes and RVOs with anti-VEGF therapy or laser therapy, and neovascular AMD patients with anti-VEGF therapy. We can also follow the progression of nonproliferative DR by identifying changes in the areas of decreased capillary perfusion before patients become symptomatic, which helps with overall diabetic management recommendations. We also use this technology to exclude neovascularization in patients with diseases that can masquerade as neovascular conditions such as macular telangiectasia type II, vitelliform macular dystrophy, central serous chorioretinopathy and inflammatory lesions.
IN PRACTICE
Currently, I use OCTA as a research tool, but we are in the process of upgrading all of our conventional OCT instruments to perform OCTA. During clinic, I image as many AMD, DR and RVO patients as possible, but I don’t always review all of the information with the patient still present. Due to the required time and effort to perform a thorough review, I only evaluate those cases in which there is an immediate diagnostic or management question. In a real-world clinical setting, I plan to follow a similar paradigm:
- Acquire OCTA scans on most of my patients. I get both structure and flow information from the same scan.
- Review the structural images on all patients. I will only review the flow images if I suspect that what I find will influence my diagnostic or clinical management.
- Bank all other scans for future reference.
READING OCTA IMAGES
Once I perform the OCTA and obtain cube scans — ranging in size from 3 mm × 3 mm to 12 mm × 12 mm depending on the disease being studied — I initially review the retinal layers and the choriocapillaris layer under the retinal pigment epithelium. When analyzing any retinal or choroidal layer, I review four images: the en face flow image from the specific layer, the en face structure image from the specific layer, the B-scan structural image and the B-scan flow image. The B-scan images should have the segmentation boundaries that correlate with the en face image. First, I always compare the flow image with the structural image. Often, analyzing a flow image on its own can be misleading unless the structural image from the same slab is viewed simultaneously. This is particularly important when decreased flow is detected. It’s important to exclude the possibility that the decrease in flow is an artifact of not having an adequate structural signal. Therefore, the four images, two en face images and two B-scans, represent the totality of the information that allows me to make a diagnosis and decide treatment (Figure 1).
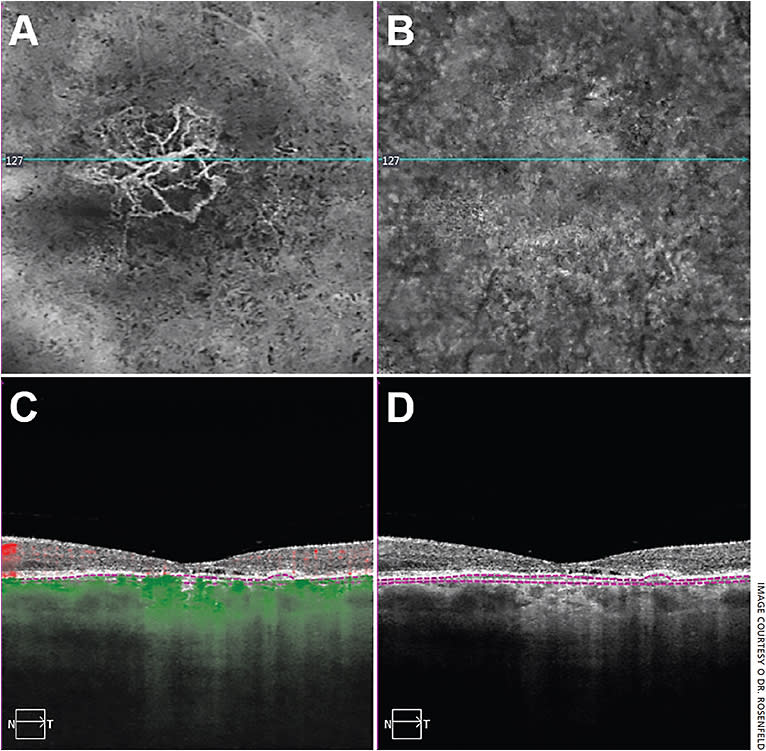
If the patient has a retinal disease, I review the total retinal flow. Then, I divide the retina into the superficial retinal flow and the deep retinal flow, which I review separately. Next, I review the outer retinal flow, which should be nonexistent because the outer retina is avascular. For diseases of the choroid, most instruments divide the choroid into different layers that allow the user to look at the flow right under the retinal pigment epithelium, which should be the choriocapillaris, and then look at the flow in different deeper layers in the choroid.
When evaluating neovascularization in AMD, it’s important to look in the outer retina for type 3 neovascularization, just above the RPE for type 2 neovascularization, and just below the RPE for type 1 neovascularization. One slab that we’ve developed that extends from the outer retina to the choriocapillaris (known as the ORCC slab) can visualize all three forms of neovascularization and is best used with an algorithm that removes the retinal projection artifacts, which can appear in any layer that includes the RPE.
CONCLUSION
OCTA is here to stay, and it’s only going to get better with faster acquisition times, wider fields of view and better algorithms to detect pathology. My advice to my colleagues: jump in now and learn the technology. At first, it can be frustrating, but once you learn how to navigate the ocular layers and interpret the images, you’ll never go back to dye-based angiography. What is most important, you’ll take better care of your patients. OM
REFERENCES
- Zhang Q, Chen CL, Chu Z, et al. Automated quantitation of choroidal neovascularization: A comparison study between spectral-domain and swept-source OCT angiograms. Invest Ophthalmol Vis Sci. 2017;58:1506-1513.
- Potsaid B, Baumann B, Huang D, et al. Ultrahigh speed 1050nm swept source/Fourier domain OCT retinal and anterior segment imaging at 100,000 to 400,000 axial scans per second. Opt Express. 2010 Sep 13;18:20029-20048.