The Early History of Laser Vision Correction
Before corneal refractive surgery as we know it today …
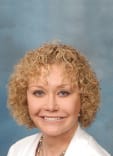
By Marguerite B. McDonald, MD, FACS
The history of laser vision correction (LVC) is by any measure a colorful one. It is packed with visionaries and pioneers whose names are now engraved in ophthalmology's heritage. Many are recognized universally in the field. A great many more also made substantial contributions to the evolution of LVC as we know it today. Some are not immediately associated with LVC, perhaps because their work was originally focused in other areas, such as physics, the military or the computer world. Some made important contributions in other areas of ophthalmology that tend to overshadow their LVC-related accomplishments. Still others saw their contributions go unpublicized or unrecognized by the courts, where battles over competing discovery claims and patent challenges have been waged frequently. Also in the mix are countless fellows, research assistants and technicians who aided lead investigation teams along the way.
The history of LVC is peppered, too, with humorous anecdotes, such as ablating leftovers from Thanksgiving dinner and performing surgery in a double-wide trailer. (More on those later.) And LVC, like so many medical innovations, faced its share of doubters. I remember during my early work with PRK in animal eyes when my cornea fellow, followed quickly by two of my technicians, abandoned ship. “This is going to bomb,” came the fellow's declaration. “It's just too depressing to work with you on this project. I quit.”
The Move Toward Laser
That particular day was several years after 1981, which was when I joined the faculty of the Louisiana State University Eye Center in New Orleans as an assistant professor, my first job after fellowship. I'd been involved in refractive surgery as a fellow at LSU Eye Center, during which time I worked with my professor, Herb Kaufman, MD, to develop epikeratophakia, a form of lamellar keratoplasty also known as the KME procedure (Kaufman McDonald epikeratophakia).
As an assistant professor, my involvement in the development of refractive surgery continued. I replaced a senior surgeon who had relinquished his position as the Prospective Evaluation of Radial Keratotomy (PERK) principal study investigator at LSU when he moved out of state. PERK had gotten under way in the early 1980s after Dr. George Waring III secured a multimillion-dollar National Eye Institute (NEI) grant to examine the safety, efficacy, and long-term effects of RK. Such a study had not been done previously despite the growing popularity of the procedure. By the time the 10-year results of the study were released, close to 250,000 RKs were being performed per year in the United States, according to the NEI. The trial was beautifully designed and very few patients were lost during follow-up.
At the 10-year follow-up, about 70% of the PERK study patients reported they did not wear corrective lenses for distance vision—impressive results indeed. However, nearly half had experienced a gradual shift toward farsightedness over the years. The hyperopic shift was so slow it likely would have gone undetected for another decade if not for the study. Nevertheless, PERK served as an impetus to find a different surgical means of correcting refractive error that would be more precise than RK with no long-term downside.
Enter the Excimer
In the 1970s, excimer lasers were being used in military communications and to etch computer chips. By 1980, two physicists in two different places—John Taboada at an Air Force base in Texas and Rangaswamy Srinivasan in an IBM research lab—had been considering the idea of using the excimer for medical purposes. James Wynne, an IBM colleague of Srinivasan, recently shared some stories from their research experiences on the LaserFest* Web site. “What really broke things open, after all the talk of what kind of tissue we would use, was that Sri [Srinivasan] brought his Thanksgiving turkey leftovers into the lab … to etch a pattern in whatever bone, cartilage or meat was on the tissue sample,” he said.
*LaserFest, a collaboration between the American Physical Society, the Optical Society, SPIE and IEEE Photonics Society, is a yearlong celebration of the 50th anniversary of the laser.
Around this same time, Stephen Trokel, MD, then an associate professor of ophthalmology at Columbia University in New York, became aware that scientists using excimer lasers in the military and in Silicon Valley were experiencing ocular side effects. He was intrigued with these observations, and wanted to understand these effects.
Dr. Trokel acquired one of the lasers and enlisted the help of physicist and optical engineer Charles Munnerlyn to adapt it for ophthalmic applications. Through experimentation on cadaver animal eyes, Trokel discovered that the excimer was capable of removing precise volumes of corneal stroma, creating extraordinarily well-controlled incisions and wedge resections with no thermal damage to surrounding tissue. He first reported these findings in a landmark paper in the American Journal of Ophthalmology in December 1983.1 When I read the paper, I called Dr. Trokel and told him I'd like to work with him. I was a fairly recent graduate of Columbia Physicians & Surgeons medical school and I'd worked briefly with him on a different project, so I knew he didn't have easy access to living animal subjects, such as rabbits and monkeys, which would be necessary for the next step of the research. At LSU, I had ready access to research animals, primates in particular. Also, primate corneas are closer to human corneas than any other corneas in the animal kingdom, so I knew they would be an excellent predictor of the excimer laser's behavior in humans.
During 1984, many discussions and planning sessions took place between Munnerlyn, Trokel and myself regarding the excimer laser experiments on plastic, cadaver animal eyes, cadaver human eyes, and living animals that would take place at LSU Medical Center in New Orleans and the Delta Primate Center in Covington, La. During this time, the research community felt the excimer laser would be best developed as a more precise way to perform RK. Several well known researchers pursued this avenue. Munnerlyn, Trokel and myself, however, decided as a team to pursue a novel approach: wide area surface ablation, which became known as photorefractive keratectomy (PRK). Eventually, time and the clinical results would show this decision to be a wise one. Thus began a very unique and interesting partnership between Drs. Trokel, Munnerlyn and me.
You Have to Start Somewhere
In many ways, the three of us were like the three legs of a stool: without the contributions of all three, LVC would never have “stood up” to reach clinical fruition.
We were the first to demonstrate that the excimer laser could directly ablate and gently change the corneal shape over a wide area, rather than being used as a scalpel to perform radial keratotomy. The results of our early animal studies of large area ablations—the concept initially conceived of by Trokel and performed by McDonald on a laser system developed by Munnerlyn—were presented at a conference in Berlin in September of 1986. After that, the “seed” had been planted. Other researchers and clinicians also helped to move LVC research forward.
Beginning in 1985, following hundreds of experiments by our threesome on plastic, cadaver animal eyes, and cadaver human tissue, I had the honor of being the first surgeon in the world to perform laser vision correction—in the form of myopic PRK—on cadaver animal and human corneas, and living rabbits and primates. The laser in those early days, to say the least, was a far cry from those in use today. It started off as a very small portable unit, about the size of a bread box. We would fire it for a few seconds at our rabbit subjects from a distance of 6-10 feet, manually crank down the diaphragm to a smaller diameter, and repeat. We must have looked like the Keystone Cops! Not to mention the resulting hyperplastic, opaque corneal scars that resulted. These scars were unexplained, though we made the “intuitive leap” that perhaps our ablations weren't smooth enough. Following much discussion, Dr. Munnerlyn added an automated diaphragm movement to the laser; instead of five diaphragm positions that were “stopped down” manually—using a crank—between shots, the new motorized diaphragm now had 40 positions, which closed automatically. The ablations became markedly smoother, and the hyperplastic scarring issue was largely behind us.
With every passing week, Dr. Munnerlyn improved the optics and performance of the laser. Dr. Trokel and I were able to influence the design of the laser from our perspective as clinicians as well. For example, we advocated for a normal operating microscope setup so the surgeons would feel comfortable, rather than the original concept of placing the patient's head in a console (“box”), with the treated eye monitored from a video screen.
An integral part of the LSU team from the very beginning was Stephen Klyce, PhD, a professor of ophthalmology at LSU Eye Center. As a corneal physiologist and biomechanical engineer, Dr. Klyce contributed to the project in many ways, particularly in the areas of corneal healing, eye fixation (a tough challenge before eye trackers), eye alignment (avoiding “pitch and yaw”), and plume evacuation. Perhaps his biggest contribution was in the area of corneal topography. By examining the topography maps of thousands of rabbit, monkey and human subjects, Dr. Klyce first described the “central tigated the impact of ablation profile and diameter on postoperative healing and higher-order aberrations.
Once we'd finally progressed to treating living monkeys at the Delta Primate Center in Covington, an unexpected opportunity arose, thanks to the selflessness of a 61-year-old patient named Alberta Cassady. She'd just been diagnosed with malignant melanoma of the conjunctiva. Knowing she was scheduled for an exenteration, she asked if anyone would like to experiment on her otherwise normal eye beforehand. So, on April 4, 1988, we rushed Mrs. Cassady past the monkey cages and sacks of feed to the operating suite at the Delta Primate Center, and that's where I performed the world's first laser vision correction procedure, a PRK, on a living human subject. We monitored her healing every day, and received the histological specimen after her exenteration 11 days later. Even though Mrs. Cassady was not a woman of means, we convinced the university to name our trailer the Alberta Cassady Laser Laboratory. Mrs. Cassady passed away 18 months later of metastatic melanoma.
Given that the laser caused no damage internally or to surrounding areas of Mrs. Cassady's cornea, the FDA permitted us to expand our efforts to blind and then partially sighted and sighted human eyes. This allowed us to further develop the perioperative protocols involving anesthesia, topical and oral medications, and postoperative care that would be essential for the success of PRK.
Where I performed our early procedures was just as primitive as how. After Mrs. Cassady's surgery, we were allowed to move our laser out of the Delta Primate Center in Covington, transport it across Lake Pontchartrain, and place it back in New Orleans on LSU Medical Center property. Our new “laser suite” became a double-wide trailer adjacent to the LSU Medical Center trash compactor. Inevitably, while we were operating, the compactor would turn on and shake the trailer. But, believe it or not, that worked in our favor. The wobbling of the trailer helped to further smooth the ablations, which led to clear corneas and accurate refractive outcomes. In 1993, as another result of my collaboration with Drs. Munnerlyn and Trokel, I had the honor of performing the world's first hyperopic ablations as well.
With our body of work, we were the first to demonstrate that the excimer laser could directly ablate and gently change the corneal shape over a wide area, rather than being used as a scalpel to perform RK as others were trying at the time. We showed that corneas healed normally and that refractive change was predictable. Dr. Munnerlyn devised the formulas for tissue removal that still serve as the basis for all excimer laser surgery. He, Dr. Trokel and Terry Clapham eventually founded VISX (which was subsequently merged with Francis L'Esperence's Taunton Technologies), the company that for years was synonymous with LVC.
LASIK Takes Center Stage
Even before the FDA granted the first approval for commercial use of PRK in 1996 for its well documented safety and efficacy, the concept began to emerge of sculpting the cornea without PRK's immediate postoperative pain and fairly slow return of vision (1 to 2 weeks). The concept came to fruition, as we know, with LASIK. Laser-assisted in situ keratomileusis (LASIK) involves two devices instead of one: the same excimer laser is used as in PRK, but the ablation is performed under an anterior hinged flap of corneal tissue that is created by a mechanical microkeratome or—more recently—a femtosecond laser.
Greek ophthalmologist Ioannis Pallikaris is credited with developing LASIK; he performed the first procedure on a human eye in 1989. Interestingly, in the same year, half a world away in Louisiana, Gholam Peyman applied for and received a patent for the same procedure. A new flurry of laser and microkeratome-related advancements and FDA approvals ensued—the mechanical microkeratomes of Jose Barraquer and Luis Ruiz, excimer laser eye trackers, and excimer laser treatment registration. The list goes on. Millions more LASIK patients were added to the ranks of those who benefited from PRK. Together, these two procedures and PTK (phototherapeutic keratectomy) have enabled surgeons to not only correct ordinary refractive error, but also help patients with corneal irregularities and reduced vision that were uncorrectable by other means.
The Evolution Continues, Mine Included
My involvement in LVC didn't end with PRK. I served as principal investigator in several LASIK clinical trials, and in 1999, as part of the Summit/Autonomous-sponsored FDA study, performed the first wavefront-guided surgeries in the United States (actually, the first outside of Germany) and the first in the world with that particular laser. Wavefront technology set LASIK on a new course. The results of that first Summit/Autonomous trial moved me in a different direction as well. As outstanding as the LASIK outcomes were, the outcomes for patients who received PRK in those studies were even better.
Based on those results, and subsequent and similar findings by other investigators, I decided that in my practice, I would perform 100% wavefront-driven surface ablations. I focused my PRK research on developing a perioperative regimen that virtually eliminated the immediate postoperative pain and greatly sped the return of vision. In addition to great results, this move came with an added bonus—no flap, no flap complications and (so far) no ectasia. Many surgeons in many countries have increasingly gone “back to the surface,” taking advantage of even newer techniques. In fact, in 2003, I became the first surgeon in North America to perform Epi-LASIK, an iteration of surface ablation that is similar to PRK but preserves the overlying epithelial layer. I will continue to adopt whatever new LVC technologies and techniques—surface or otherwise—are shown to provide the best in safety and efficacy for my patients. Many such opportunities, in the form of femtosecond lasers, corneal cross-linking, intracorneal inlays, and others, are already presenting themselves.
In Their Own Words The Past, Present and Future of LASIK as Viewed by its International Pioneers |
---|
Theo Seiler, MD, Phd Many developments in excimer laser surgery have taken place in Europe during the past 20 years. Phototherapeutic keratectomy, aspheric ablation profiles, scanning spot systems, wavefront-guided treatments, topography-guided treatments and even femtosecond laser treatments started in Europe (Hungary, Germany). LASIK as a procedure was proposed in Russia and brought to a clinical level in Greece and Italy. In essence, nearly all steps of development of modern LASIK have been performed outside of the United States. With the advent of more precise femtosecond lasers, I hope we will be able to improve the excimer laser within the next few years and perform a clinically satisfying “all femto-LASIK.” Lucio Buratto, MD The story of LASIK began many years ago. The technique was invented by Josè-Ignacio Barraquer in Colombia, South America. It was then developed and perfected in Europe by Jorg Krumeich, MD, in South America once again by Antonio Ruiz, MD, and, finally, in Europe by Dr. Theo Seiler, Joannis Pallikaris, MD, and myself. But what about the United States of America? During these development phases, the American surgeons were simply observing the technique's ground-breaking evolution. They had the advantage of not having to pay the bill associated with these major surgical steps forward (in reference to the cost of the surgical complications). As a result, American surgeons found themselves in a position to provide their patients with a tried and tested technique, which translated into extremely positive surgical results. In more recent years however, the fields of technology and ophthalmology in America have also driven the optimization of this type of surgery, with a major contribution to technique as it is today. LASIK is now the elective refractive technique for myopia, hyperopia and astigmatism in more than 90% of refractive procedures. The standard procedure involves the use of large zones for smooth ablations delivered by small beam, flying-spot, tracking lasers. The bar has been raised further by the use of more sophisticated lasers to perform custom ablations, tailored more specifically to a patient's optical defects, which are determined by corneal topography and wavefront analysis of the entire optical system. In the current standard operating technique, the mechanical blade previously used to create the cut is replaced with a femtosecond laser, which provides greater safety, precision and flexibility. So what does the future hold? A better understanding of the corneal aberrations and corneal biomechanics of the eye. This will mean improved correction of the preexisting and induced aberrations. And, in terms of the laser, the excimer laser will be used less with greater use of the femtosecond laser. Michael Mrochen, PhD Current customized excimer laser ablations using LASIK are based on corneal topography, whole eye wavefront or corneal wavefront technology. Customized ablation, whether topography-based or ocular wavefront-based, has been developed to address disadvantages of conventional sphero-cylindrical ablation. However, the simplified formulae from paraxial optics fail to consider the multiple lens structure of the eye. Existing customized methods offer a higher individualization of the ablation profile, but still fail to address all optical errors of the eye, resulting in a clinical outcome that is generally poorer than expected. These custom ablation profile algorithms are based on values obtained using just one diagnostic method, either topography or ocular wavefront and, as such, still depend on approximations. Even the planning of refractive treatments using wavefront technology, which is known to measure the entire optical characteristics of the eye, is based upon an approximation. It is presumed that the total measured wavefront aberration of a multi-lens system can be compensated by applying the corresponding correction profile to a single refractive surface of the system without further adjustments of the profile, which is not exactly the case. The highest possible accuracy in ablation profile and IOL planning can only be achieved by the consideration of all structures of the patient's eye. An exact computer model of the patient's eye must be generated from the measurements. After an individualized model of the patient's eye has been created, it can be used for various treatment planning tasks. This includes corneal ablation profile planning, intraocular lens calculation or combinations of both. The multi-lens aspect of the eye will automatically be taken into consideration. All of the optimization processes must be executed iteratively until the desired quality is reached. It is the iterative nature of the optimization that ensures superior accuracy over traditional planning methods. In order to plan a laser ablation profile, the eye model will be used to calculate the optimal corneal front surface for the desired optical quality. The calculation is performed in two steps: 1) 3-D ray tracing from the retina to the cornea and 2) Iterative calculation of the ideal corneal front surface. The optimized ablation profile finally results from the difference between the preoperative corneal topography and the calculated ideal front surface of the cornea. In contrast to the traditional wavefront ablation profiles, which are directly calculated from the measurement, the optimized profiles already compensate for the previously mentioned multilens effect. |
Dr. McDonald is in practice with Ophthalmic Consultants of Long Island, a multispecialty eyecare group serving patients in seven locations. She is a clinical professor of Ophthalmology at NYU Langone Medical Center and an adjunct clinical professor of Ophthalmology at Tulane University in New Orleans.
REFERENCE 1. Trokel SL, Srinivasan R, Braren B. Excimer laser surgery of the cornea. Am J Ophthalmol. 1983;96(6):710-715. |